Multi-messenger astronomy
Over the last few years, a new area of astronomy has started to emerge. It's an area that I'm very interested in and have been working on as much as my regular duties on the Swift team will allow. It's called ‘multi-messenger’ astronomy. Since I think it's awesome, I thought I'd write a little article explanining why.
What is multi-messenger astronomy?
When we talk about ‘astronomy’ we normally mean looking at stars, galaxies etc. to study how the universe works. In this context ‘looking’ may mean with our eyes or with telescopes, radio dishes, satellites and so on; but what we mean is that we are going to detect light coming from space. Light, be it radio light, optical light, gamma-ray light or anything in between, is the messenger we use to study the cosmos. Or at least it was. Over recent years, technological developments have enabled us to detect other stuff, or messengers, from space, such as neutrinos or gravitational waves. The idea of multi-messenger astronomy is to combine these different messengers.
But why do you want to do this?
Lots of reasons! Whenever we find a new way of looking at things, we learn new stuff. Until around a century ago, not only were we limited to light, but to that tiny part of it that our eyes can see. As technology allowed us to detect other wavelengths, we learned more about the objects we knew of, and discovered brand new things, like pulsars, black holes and gamma-ray bursts. So as we learn to observe with neutrinos and gravitational waves, we expect similarly to find new things and to learn more about objects we already know of.
A good example of the latter is what we sometimes call, ‘Compact Binary Coalesence.’ This is where we have two compact objects, such as neutron stars or black holes, in orbit around each other (a ‘binary system’). As they orbit they give off gravitational waves. These are far too weak for us to detect with current facilities, but strong enough to take energy away from the binary; that is, they cause the stars to spiral in towards each other. The closer the stars get, the quicker they orbit which means that they radiate more energy as gravitational waves, causing them to fall in even closer and so radiate faster and so on. Eventually they will spiral in and coalesce. Just before this coalscence, the gravitational waves are strong enough for LIGO and Virgo to detect.
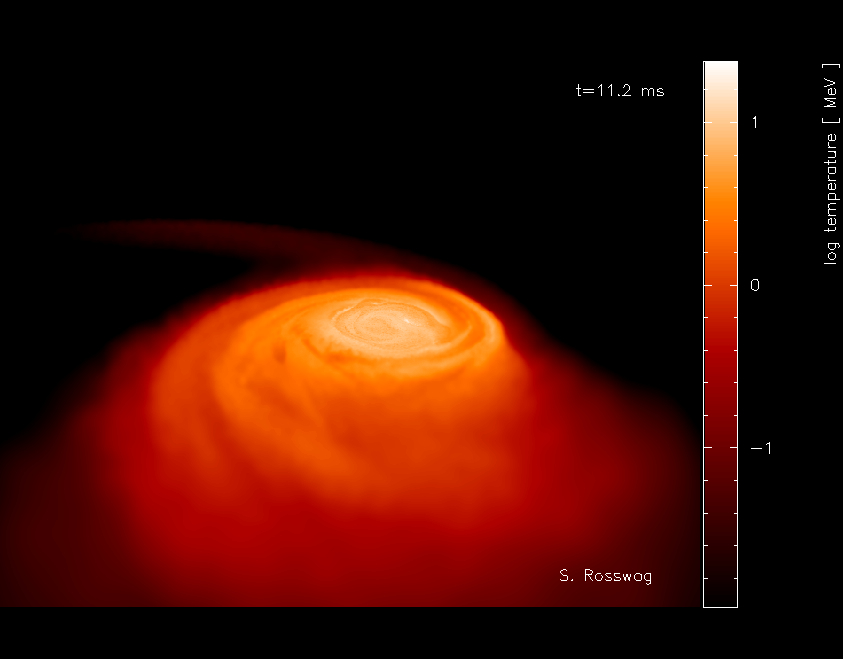
Dynamical simulation of a binary neutron star merger, showing all the material ripped off the stas as they merge. Credit: Stephan Rosswog.
We (think we) already know quite a bit about compact binary coalescence. We believe that the merger of two neutron stars, or maybe a neutron star and a black hole, are what give rise to short gamma-ray bursts (GRBs). We also think that when a neutron star merges with another neutron star or a black hole, it will be torn apart, leading to a debris cloud of very neutron-rich material. This debris cloud is a great candidate to be the place where many of the heavy elements in the universe are synthesised, via something called ‘rapid neutron capture’ or the ‘r-process’ (Stephan Rosswog's website contains some great movies of simulations of this; the still to the right comes from one of his movies).
The problem is, most of what we know, or think we know, we have learned indirectly. We don't see neutron stars merging, we see a flash of gamma rays and then a fading afterglow both of which comes from material speeding away from the merger. From studying these things, their nature and where they occur, we infer that the cause was a compact binary coalescence. But with multi-messenger astronomy we can join the dots. LIGO and Virgo do ‘see’ the compact objects merging, the gravitational waves come directly from the binary merger. From these we can work out the details of what merged: what mass were they? How fast were they spinning? Then we can combine this with the light that we see to find out whether our models were correct, and to start to pin down the details of the physics that's going on. Also, using gravitational waves we can detect objects that we miss when we search with light: those where our viewing angle to them means we don't see a GRB. By then searching for light from these events, which are nearby because LIGO and Virgo can't detect distant object, we can find emission from radioactive decay of those r-process elements (we call this a kilonova. Or a macronova. Or sometimes a mini-supernova. Yeah, I know). And not only does this multi-messenger approach let us find these things, but it gives us the complementary information to study them in detail, to see whether movies like those I linked to above are right or not, and to understand where all those heavy elements in the universe actually came from.
Of course, this is one example of the science that multi-messenger astronomy enables, and I chose it because it's what I'm interested in. But there are plenty more. Supernovae give off light, but they should also give off gravitational waves and neutrinos. Again, these complement each other. The light we see from the supernova tells us about the nuclear reaction and decays that take place in the matter blown off by the explosion, while the gravitational waves tell us what actually went on at the core of the star (which probably became a neutron star or black hole) and the neutrinos teach us about the nuclear physics going on in the collapsing core.
And there are the unknowns. Just like the birth of radio astronomy led to the discovery of pulsars, we should expect to find new things using these new messengers. Indeed, we alread have. The IceCube neutrino observatory has detected neutrinos with really high energies and we don't know where they came from! The first gravitational wave detections were of high mass (30 times the mass of the Sun) black holes merging. We didn't even know that 30 solar mass black holes existed before! And explaining how you manage to make one is a challenge; how to get two in a binary, and close enough to each other that the merge, is a real headache. As the field of multi-messenger astronomy matures, who knows what else we will discover?
So what do you do?
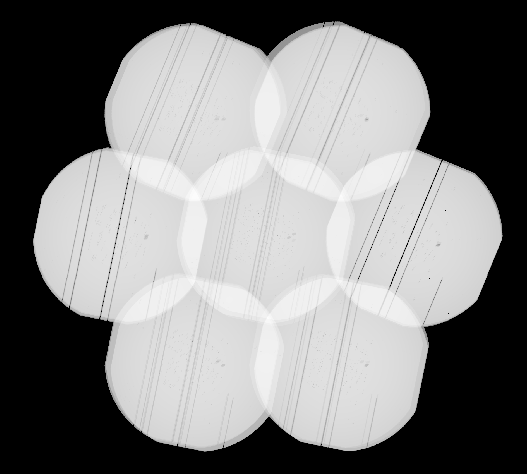
Example of a Swift observation of a neutrino event. The colours indicate the about of exposure (white is more). Here 7 overlapping fields were used. The black streaks are parts of the CCD damaged by a micrometeroid impact.
Indeed, am I just excited about this, or actually doing something? The latter, definitely. For a number of years now, Swift has been responding the possible neutrino detections, observing the area where the neutrinos came from with the X-ray and UV telescopes, looking for some kind of event in light that may have caused the neutrinos. This is not as easy as you may think, because we don't know exactly where the neutrinos came from and we can't fit the whole likely origin in one field of view (see here for a discussion of locating objects in space). Instead we have to do a sort of mosaic, where we point the telescope in a pattern (see the image to the left) to cover the area the neutrinos probably came from. And the problem with this is that, when you look at a large area of sky with our X-ray telescope, you generally find a bunch of objects that have never been seen before (because no-one's looked in that direction with a sensitive X-ray telescope before). So how do you work out which, if any, is the source of the neutrinos?
This problem is even worse for gravitational waves. It's really hard to tell accurately where they came from, and for the events that LIGO have published so far, we'd have to look in hundreds or thousands of different places with the X-ray telescope to cover the area of sky from which the waves could have originated. And of course this means we'll find loads of objects and have to work out which one (if any) gave off the gravitational waves!
My focus so far has been on these problems. I've looked into ways of reducing the amount of sky we have to search, and produced methods of combining the information about where the gravitational waves came from, with information about where nearby galaxies are — and how complete with think our lists of those galaxies are — to do this. I've made software to optimise when and where we point Swift to maximise our chances of looking in the right place at the right time. I've made tools to analyse the X-ray data automatically to try to work out if we've found anything that looks like it might be the aftermath of a compact binary coalescence event. If you're interested you can look at my publication list for some of my recent works on this (the 'Evans et al.' papers from 2015 and 2016). If and when Swift detects light from a gravitational wave event, I will be spending what time I can studying it and writing it up. At present, because I'm employed to work on Swift, I have limited time available to spend doing this awesome science, but as you can imagine with this field just maturing, I'm doing what I can to find myself a dedicated research role to work on this sort of thing!
Further information: From swift.ac.uk | From NASA.